BACKGROUND
Oregon State Pen.
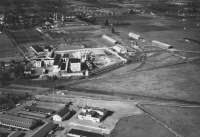
The Oregon State Penitentiary (OSP) is approximately 306.3 acres and is located at 2605
State Street, Salem, Oregon, approximately one-half mile west of Interstate Highway 5 in
Salem, Marion County, Oregon [Figure ES-1,
SECOR, 1998]. The state of Oregon has owned and operated the subject property as the
Oregon State Penitentiary since approximately 1865. Included on the OSP site are prison
inmate living buildings and working facilities. The working facilities include the Motor
Pool, the Industries Metal Shop, the Vocational Training Building, the Physical Plant
Building, and the Industries Laundry facility. The Industries Laundry facility is located
in the northwest quadrant of the OSP property and currently includes approximately 28,880
square feet of building space. Although not verified, the SECOR reports suggest that the
source of groundwater contamination is associated with the past operation of the
Industries Laundry facility. Dry cleaning and laundering operations have occurred at the
site since late 1950s.
Oregon State Pen.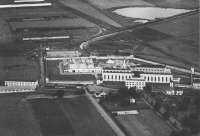
In this study the results of a preliminary contaminant fate and transport analysis
conducted at the OSP, Salem, Oregon is summarized. In this analysis, the Analytical
Contaminant Transport Analysis System (ACTS) software is used. The purpose of this work is
to assess the distribution of the Trichloroethene (TCE) and the Tetrachloroethene (PCE),
determine the potential groundwater exposure pathways of TCE and PCE at the OSP site and
provide recommendation for further studies. The site specific data are obtained from site
investigation reports prepared by SECOR (1998) and
SEACOR(1994).
Identification of Contamination Sources at the
OSP Site
The groundwater samples obtained from the on-site OSP water supply wells were analyzed
in October 1989. The sampling results indicated the presence of various Halogenated
Volatile Organic (HVO) compounds, including TCE, PCE, dichloroethene (DCE), and vinyl
chloride (VC). The highest concentrations were detected in the old OSP water supply Well
No. 3 located at the northwest corner of the OSP site. A Preliminary Assessment at the OSP
site in 1991 identified the discharge of non-contact cooling water used by the facility
dry cleaning operation as a potential migration pathway for PCE to subsurface soil and
groundwater. The cooling water was discharged to the storm sewer on the east side of the
Industries Laundry building. Site investigation results show that PCE and TCE
concentrations were detected in monitoring wells MW-1, MW-2, MW-2I, MW-2D, MW-5, MW-7,
MW-8, and MW-9. The highest contaminant concentrations were consistently detected in
groundwater samples collected from MW-5, which is located at the east side of Industries
Laundry building.
Groundwater was sampled from the shallow aquifer zone, the intermediate aquifer zone,
and the deep aquifer zone. The highest HVO concentrations were observed in the shallow
zone at well MW-5, which is located just east of the present Industries Laundry facility.
The TCE concentration up to 600 µg/L has been detected from MW-5. The distribution of TCE
concentration detected in July, 1996 based on the shallow monitoring well data are
illustrated in Figure
1. Similarly, the PCE concentration as high as 17,000 µg/L has also been detected at
MW-5 in the shallow aquifer. In addition, PCE concentrations of 930 µg/L, 1,000 µg/L,
and 220 µg/L have been measured in down gradient monitoring wells MW-2, MW-9, and MW-10,
respectively, Figure
2. The major HVO release from the Industries Laundry facility is PCE and the presence
of TCE is most likely due to the degradation of PCE. The analytical results of discrete
groundwater samples collected during the drilling of the monitoring wells indicate large
variation of HVO concentration vertically.
Hydrogeologic Characteristics of the OSP Site
The primary aquifer at the OSP site is the Troutdale Formation. The Troutdale formation
ranges in thickness from 120 to 260 feet at the site. There are three groundwater zones in
the Troutdale aquifer: the shallow zone, intermediate zone and the deep zone (Figure ES-5,
SECOR, 1998). The shallow zone in the OSP study area is defined as the uppermost
portion of the saturated zone of the Troutdale Formation with the bottom at approximately
30 to 40 feet below ground surface. The intermediate zone extends from the bottom of the
shallow zone to approximately 100 feet below ground surface. Between the shallow zone and
the intermediate zone is a gravelly clay layer of low hydraulic conductivity. The deep
zone of the aquifer extends from the bottom of the intermediate zone to approximately 130
feet below ground surface. Similarly there is a gravelly clay layer of low hydraulic
conductivity between the intermediate zone and the deep zone. Within the vicinity of the
OSP facility, the bottom of the deep zone corresponds to a low hydraulic conductivity
layer overlying the Columbia River Basalt (CRB).
Based on water level data collected from the groundwater monitoring wells, the inferred
groundwater flow direction in the three groundwater zones of the Troutdale Formation is
oriented in a northwesterly direction. The average groundwater level gradients are 0.006
ft/ft, 0.004 ft/ft, and 0.0045 ft/ft for the shallow, intermediate, and deep zones
respectively at the OSP site study area (SECOR, 1998). The static water level data
collected from the OSP site also indicated the existence of vertical gradients between the
shallow, intermediate, and deep zones of the Troutdale Formation. A pumping test was
conducted at the Suburban East Salem Water District (SESWD) LaBranch Street Well No. 3-A.
This test indicated the average transmissivity value of the Troutdale Formation is
approximately 3.4 ft2/min with a storativity of 3.3 x 10-4. In addition to the SESWD pump
test, limited aquifer recovery tests were conducted at off-site wells MW-11M and MW-12M
during drilling to determine more site-specific values of transmissivity. Analyses of the
recovery test data indicated transmissivity values ranging from 0.0034 to 0.016 ft2/min.
Assumptions and Simulation Parameters
The hydrogeologic setting at the OSP site is rather complicated. There are three
groundwater flow zones in the Troutdale Formation. Field investigations at the site
indicate the existence of vertical hydraulic gradients between shallow, intermediate, and
the deep aquifer zones. There are flow interaction between these zones and the groundwater
flow direction among these zones may vary spatially. Based on the results of field
investigations (SECOR,
1998), the Industries Laundry building is the potential location of the contaminant
source. In the analysis presented in this report, the location of the monitoring well MW-5
will be treated as the approximate location of the contaminant sources. The x- and y-
coordinate directions selected in the ACTS simulations are shown in Figure 1 and Figure 2. The
x-axis direction is selected in the main groundwater flow direction which is towards the
Northwest and the coordinates of the monitoring well MW-5 is defined as the origin of the
coordinates. In our preliminary investigation of the PCE and TCE fate and transport
conditions at the site, the groundwater flow is assumed to be steady and the vertical flow
interaction between different aquifer zones is neglected. The contaminant simulations are
done separately for PCE and TCE for the shallow zone and the chemical interaction between
PCE and TCE is also neglected. The parameters used for one-dimensional and two-dimensional
PCE and TCE fate and transport simulations are given in Table 1 and the
Monte Carlo simulation parameters are shown in Table 2.
Evaluation of TCE and PCE Fate and Transport at
OSP
TCE and PCE fate and transport simulations are conducted using the ACTS screening
models (Aral,
1998). For each contaminant, simulations are done using one-dimensional analytical
transport model and two-dimensional analytical transport model separately. To evaluate the
effect of uncertainty of the parameters on the TCE and PCE fate and transport, Monte Carlo
simulations are also performed for each contaminant. The evaluation of the results of TCE
and PCE fate and transport analysis are summarized in the following sections.
TCE and PCE Fate and Transport
Simulations For the one-dimensional model, the flow direction is in the x-axis
direction, the source of TCE and PCE contamination is along the y-axis at x = 0, Figure 1. The
PCE concentration at the source location is assumed to be 17,000 µg/L, which is the
highest PCE concentration detected at the OSP site. Similarly, the TCE concentration at
the source location is assumed to be 600 µg/L, which is also the highest TCE
concentration detected at the OSP site. Since lateral dispersion is neglected in
one-dimensional models, the simulated TCE and PCE concentrations are conservative and
generally larger than their field measurements. However, one-dimensional simulation
results may provide useful information on the upper limit of the contaminant
concentrations.
The aquifer and chemical parameters used in the TCE and PCE fate and transport
simulations are shown in Table 1. As shown
in this table, the average groundwater flow velocity (Vx = 0.67 ft/day), the longitudinal
dispersion coefficient (Dx = 67 ft2/day) are selected as representative values. The
contaminant half-life is assumed to be very large for both PCE and TCE representing
conservative contaminant. The retardation coefficient for PCE is 1.979 and that for TCE is
1.409. The difference between these retardation coefficients will result in different
transport velocities for PCE and TCE. The travel times for PCE and TCE contamination
to migrate to selected locations at the site are summarized in Table 3 and Table 4
respectively.
The OSP property boundary is about 1140 ft down-gradient to the source at the
Industries Laundry building. As shown in Table 3 and
Figure 4, TCE concentration at the OSP property boundary may be reached to the level of
1%, 5%, 10% and 20% of the TCE source concentration in approximately 2.3, 3.1, 3.7, and 5
years respectively. The travel time for PCE to reach the same percent source concentration
levels at the OSP property boundary is larger. It takes approximately 3.2, 4.3, 5.1, and 7
years for PCE at the OSP property boundary to reach 1%, 5%, 10% and 20% of the source
concentration respectively, Table 4. The
numerical results also show that it takes about 7 years for TCE and 10 years for PCE to
reach 10% of their source concentration level at the 24th street location. Based on the
one-dimensional simulation results, the TCE plume will migrate a half mile distance in
approximately 10 years and a one mile distance in approximately 22 years. As should be
expected, the PCE plume migrates slower than the TCE plume. The PCE front will migrate a
half mile distance in approximately 13 years and a one mile distance in approximately 30
years. The Willamette River is approximately 10,400 ft down-gradient from the source
location. Based on one-dimensional simulation results, TCE concentration at the river will
be 1%, 5% and 10% of the source TCE concentration in approximately 42, 47, and 49 years, Table 3. It takes
approximately 66 years for PCE concentration at the river to reach 5% of PCE source
concentration, Table
4.
The parameters used in two-dimensional fate and transport simulations are similar to
that used in one-dimensional simulation models. The groundwater flow direction is still in
the x-axis direction and the groundwater velocity in the y-direction is assumed to be
zero. The aquifer is assumed to be 1000 ft wide in the y-axis direction. The central point
of the contaminant source is assumed to be the location of monitoring well MW-5 and the
length of the contaminant source is assumed to be 50 ft along the y-axis. In the
two-dimensional models, the lateral dispersion of the TCE and PCE plume is taken into
account. The lateral dispersion coefficient is selected to be 10% of the longitudinal
dispersion coefficient, which is commonly used in the literature. The results of the
two-dimensional TCE and PCE fate and transport simulations are presented in the report
submitted to ATSDR. Similar to one-dimensional models, the travel time for PCE and TCE to
migrate to selected locations are summarized in Table 3 and Table 4
respectively.
Based on the two-dimensional simulation results, TCE concentration at the OSP property
boundary will reach 1%, 5%, and 10% of the source TCE concentration in approximately 2.6,
4.7, and 7 years respectively, Table 3. The TCE
concentration at the OSP property boundary is less than 20% of the source TCE
concentration due to retardation and lateral dispersion of TCE contamination for very
large travel times. As shown in Table 4, it takes
approximately 4.2, 6.5, and 9.5 years for PCE concentration at the OSP property boundary
to reach 1%, 5%, and 10% of the source PCE concentration respectively. Similar to TCE, the
PCE concentration at the OSP property boundary is also less than 20% of its source
concentration for very large travel times. The approximate travel times for the TCE plume
to migrate to the 24th street area, half mile, and one mile down-gradient locations from
the source are approximately 6.5, 10, and 24 years respectively, Table 3. The
travel times for the PCE plume to reach these locations are larger. It takes approximately
9, 13, and 34 years for the PCE plume to reach the 24th street area, half mile, and one
mile down-gradient region respectively, Table 4. As shown
in Table 3,
the TCE concentration at the Willamette River will reach 1% and 5% of the source TCE
concentration in approximately 53 and 76 years respectively. The PCE concentration at the
Willamette River will be 1% of the source PCE concentration in about 74 years. It will
take more than 100 years for the PCE concentration at the Willamette River to reach 5% of
the source concentration, Table 4 and
Figure 12. The TCE and PCE concentrations at the Willamette River is less than 10% of
their source concentration for travel times larger than 100 years.
The TCE and PCE concentrations and the travel times obtained from two-dimensional
simulations are smaller than those obtained from one-dimensional simulations. This is
mainly due to lateral spread of the plume in two-dimensional application. Based on the
results of one- and two-dimensional simulations, the travel time for the TCE plume to
reach the OSP property boundary is approximately 2.3 to 2.6 years and that for the PCE
plume is approximately 3.2 to 4.2 years. The time for the TCE plume to migrate to the 24th
street area is approximately 5.6 to 6.5 years and that for PCE plume is approximately 7.2
to 9.2 years. The time for the TCE plume to migrate to the Willamette River are
approximately 42 to 53 years and that for PCE would range from 60 to 74 years. These
simulated travel times are close to 60 to 80 years that are estimated by SECOR (1998).
However, the simulated travel times in this preliminary investigation are smaller than the
travel times estimated in SECOR (1998).
This is due to consideration of longitudinal dispersion of the plumes, which generally
increase the transport velocity of the plumes. It is also shown in the results that the
TCE plume moves faster than the PCE plume. This is because the retardation coefficient for
the PCE is larger than that for TCE at the OSP site.
Monte Carlo Simulations of TCE and PCE Fate and
Transport Analysis
Monte Carlo simulation could be used to evaluate the effect of uncertainty of the
parameters used in evaluation of TCE and PCE fate and transport on the results. The range
of parameters used in the Monte Carlo simulations are shown in Table 2. For this
preliminary study, the uncertainty of the retardation coefficient, the pore groundwater
velocity, the longitudinal dispersion coefficient, and the lateral dispersion coefficient
are simulated. The groundwater flow velocity is assumed to follow the normal distribution.
Estimates for the mean minimum, and maximum velocity are 0.67, 0.54, and 0.80 ft/day,
respectively. The variance is assumed to be [10% × (0.80-0.54)]2 = 0.0007. The
longitudinal dispersion coefficient and the lateral dispersion coefficient are also
assumed to follow the normal distribution. Estimates for the mean, minimum and maximum
values of the longitudinal dispersion coefficient are 67, 47, and 87 ft2/day,
respectively. The variance of the longitudinal dispersion is assumed to be 16. Estimates
for the mean, minimum and maximum values for the lateral dispersion coefficient are 6.7,
4.7, and 8.7 ft2/day, respectively. The variance of the lateral dispersion coefficient is
assumed to be 0.16. The retardation coefficients are also assumed to follow the normal
distribution. The mean, minimum and maximum values of the retardation coefficient of TCE
contamination are 1.409, 1.0, and 2.0 respectively. The variance of the retardation
coefficient of TCE is assumed to be 0.01. For PCE, those ranges are 1.979, 1.0, and 2.5
respectively with the variance to be 0.023. The Monte Carlo simulation results for TCE are
summarized in Table
5 and that for PCE are summarized in Table 6. All of
these simulations are performed for two dimensional models.
Base on the Monte Carlo simulations of TCE fate and transport, TCE contamination is
very unlikely to contaminate the area outside of the OSP property in 2 years. The
Probability of TCE concentration at the OSP property boundary exceeding 1% of the source
TCE concentration in 2 years is about 0.26%. However, the TCE will contaminate the OSP
property boundary area in 5 years. The probability of TCE concentration exceeding 5% of
the source TCE concentration in 5 years is 100% and that exceeding 10% of the source TCE
concentration is 51.2%. TCE contamination will migrate to 24th street area in 10 years.
The probability of TCE concentration exceeding 5% of the source TCE concentration at 24th
street in 10 years is 100% and that exceeding 10% of the source TCE concentration is 1.6%.
The TCE plume will migrate one mile down-gradient to the source location in 20 to 30
years. TCE may migrate to the Willamette River in 40 to 50 years. The TCE concentration at
the Willamette River will be 1% of the source TCE concentration in approximately 40 years
with the probability of exceedance to be 97% and 5% of the source TCE concentration in 60
years with the probability of exceedance to be 97.4%.
The results of Monte Carlo simulations of PCE fate and transport are very close to that
of TCE. As shown in Figures 25 through 27 of the report submitted to ATSDR, it is very
unlikely that PCE will migrate to the area down-gradient to the OSP property boundary in 2
years, the probability of PCE concentration at the OSP property boundary exceeding 1% of
the source PCE concentration in 2 years is about 0.22%. However, PCE will contaminate this
area in 5 years, the probability of PCE concentration exceeding 5% of the source PCE
concentration in 5 years is 96.6%. PCE will also migrate to the 24th street area in 10
years. The probability of PCE concentration exceeding 5% and 10% of the source PCE
concentration at 24th street in 10 years are 100% and 1.6% respectively. PCE will migrate
one mile down-gradient from the source location in 20 to 30 years, but it is not likely to
migrate this far in 15 years. The probability of PCE concentration exceeding 1% of the
source PCE concentration at the one-mile down-gradient region in 15 years is approximately
0% and that in 20 years is 99.9%. Similar to TCE, the PCE plume may contaminate the
Willamette River region in approximately 40 to 50 years. The probability of PCE
concentration exceeding 1% of the source PCE concentration at the Willamette river in 40
years is 96.9%.
The maximum and minimum TCE and PCE concentrations at the steady state or at very large
travel times obtained from Monte Carlo simulations are a function of distance
down-gradient to the contaminant source. The simulated maximum and minimum TCE and PCE
concentrations are shown in Figures 37 and 38 respectively in the report submitted to
ATSDR. As expected, larger the distance down-gradient to the contaminant source results in
smaller TCE and PCE concentrations. As shown in these Figures, the maximum and minimum TCE
and PCE concentrations at the Willamette River are approximately 5% of their source
concentration at very large travel times.
CONCLUSIONS
Based on our preliminary evaluation of contaminant fate and transport conditions at the
OSP, Salem, Oregon using the Analytical Contaminant Transport Analysis System (ACTS)
software (Aral,
1998), the following conclusions may be reached:
i. Data collected at the field suggest that the source of groundwater
contamination is the past operation of the Industries Laundry facility at the OSP site and
source contamination may have occurred as early as late 1950s (SECOR, 1998).
ii. Data collected at the OSP site show that groundwater in the
shallow aquifer at the OSP property and downstream to the OSP property boundary has been
contaminated by TCE and PCE contamination, Figure 1 and Figure 2.
iii. Data collected at the field indicate that the PCE plume
concentration is much higher than the TCE plume concentration, Figures 1 and 2. For
instance, the maximum PCE concentration detected at the OSP site is approximately 17,000
µg/L and the maximum TCE concentration is approximately 600 µg/L. This may be an
indication that PCE is the precursor chemical released into the environment and the
presence of TCE is mostly due to the degradation of PCE (SECOR, 1998).
iv. Simulation results show that it takes less than 5 years for TCE
and PCE contamination to migrate out of the OSP property boundary in a down-gradient
direction from the Industries Laundry building.
v. Based on our simulation results as well as the field investigation
performed by SECOR(1998), shallow groundwater at 24th street area which is downstream to
the Industries Laundry building has been contaminated. Simulation results show that it
takes less than 10 years for TCE and PCE contamination to migrate to the 24th street area.
vi. Simulations results indicate that it takes 20 to 30 years for TCE
and 30 to 40 years for PCE contamination to migrate one mile downstream of the Industries
Laundry building. Based on the observation that dry cleaning and laundering operations
have occurred at the site since late 1950s and based on our simulation results, PCE and
TCE plume would have reached the area one mile downstream of the source location at the
present time. However, there is no field evidence that PCE or TCE plume have migrated this
far. This may be because of the fluctuations in the natural piezometric head gradients and
the associated change in groundwater flow velocity magnitude and directions. This may also
be due to the pump and treat system implemented at the site during the last 40 years. It
should be mentioned that if no corrective action was implemented, TCE and PCE
contamination would have reached the area one mile downstream from the source.
vii. If no corrective action is taken, it will take 50 to 60 years for
TCE and PCE plumes to reach and contaminate the Willamette River through the shallow
groundwater flow pathway. Since contaminants has been released to groundwater since late
1950s, TCE and PCE contamination may reach the Willamette River approximately during the
year 2020 if no corrective action is implemented.
viii. PCE is the primary contaminant released to the environment at
the OSP site and TCE might be the results of PCE degradation. However since TCE
contamination migrates faster than PCE contamination, TCE plume may move ahead of the PCE
plume and TCE should also be included into the future evaluation of contaminant fate and
transport analysis and field studies at the OSP site.
It should be mentioned that the preliminary evaluation of TCE and PCE fate and
transport analysis at the OSP site is based on several simplifying assumptions. These are:
(i) the groundwater flow is steady; and,
(ii) the flow in vertical directions between different aquifer layers are neglected.
The contaminant transport simulations are done separately for PCE and TCE for the
shallow zone and the interaction between PCE and TCE are also neglected. The fluctuations
of groundwater flow velocity and direction change as well as flow interaction between
different aquifer layers may affect fate and transport conditions at the OSP site
significantly. Also degradation of PCE may affect PCE and TCE concentrations at the site.
Further investigation of the effect of groundwater table fluctuation and the flow
interaction between different aquifer layers, as well as the analysis of the effect of
chemical interaction between these two species on the fate and transport processes at the
OSP site is strongly suggested.